

I operated the bench-scale isMBfR system in 7 different conditions to evaluate its nitrate-removal performance. When I supplied H2 with the isMBfR (stages 1 - 6), I observed at least 70% nitrate removal, and almost all of the denitrification occurred in the "MBfR zone." When I stopped the H2 supply in stage 7, the nitrate-removal percentage immediately dropped from 92% (stage 6) to 11% (stage 7). Denitrification raised the pH of the bulk liquid to ~ 9.0 for the first 6 stages, but the high pH did not impair the performance of the denitrifiers. Microbial community analyses indicated that DB were the dominant bacteria in the "MBfR zone," while photosynthetic Cyanobacteria were dominant in the "photo-zone".
I derived stoichiometric relationships among COD, alkalinity, H2, Dissolved Oxygen (DO), and nitrate to model the nitrate removal capacity of the "MBfR zone." The stoichiometric relationships corresponded well to the nitrate-removal capacity for all stages expect stage 3, which was limited by the abundance of Denitrifying Bacteria (DB) so that the H2 supply capacity could not be completely used.
Finally, I analyzed two case studies for the real-world application of the isMBfR to constructed wetlands. Based on the characteristics for the wetlands and the stoichiometric relationships, I designed a feasible operation condition (membrane area and H2 pressure) for each wetland. In both cases, the amount of isMBfR surface area was modest, from 0.022 to 1.2 m2/m3 of wetland volume.


Widespread application of AWC is currently limited because water production, energy requirement, best technology, and water quality are not parameterized. I developed a geospatial climatic model for classical passive solar desiccant-driven AWC, where water vapor is adsorbed onto a desiccant bed at night, desorbed by solar heat during the day, and condensed. I concluded passive systems can capture 0.25–8 L/m2/day as a function of material properties and climate, and are limited because they only operate one adsorption-desorption-condensation cycle per day. I developed a thermodynamic model for large-scale AWC systems and concluded that the thermodynamic limit for energy to saturate and condense water vapor can vary up to 2-fold as a function of climate and mode of saturation.
Thermodynamic and geospatial models indicate opportunity space to develop AWC technologies for arid regions where solar radiation is abundant. I synthesized photothermal desiccants by optimizing surface loading of carbon black nanoparticles on micron-sized silica gel desiccants (CB-SiO2). Surface temperature of CB-SiO2 increased to 60oC under solar radiation and water vapor desorption rate was 4-fold faster than bare silica. CB-SiO2 could operate >10 AWC cycles per day to produce 2.5 L/m2/day at 40% relative humidity, 3-fold more water than a conventional passive system.
Models and bench-scale experiments were paired with pilot-scale experiments operating electrical desiccant and compressor dehumidifiers outdoors in a semi-arid climate to benchmark temporal water production, water quality and energy efficiency. Water quality varied temporally, e.g, dissolved organic carbon concentration was 3 – 12 mg/L in the summer and <1 mg/L in the winter. Collected water from desiccant systems met all Environmental Protection Agency standards, while compressor systems may require further purification for metals and turbidity.

In order for microalgae to be a cost-effective renewable energy source, a high CO2-transfer efficiency (CTE) is necessary. Using hollow-fiber membranes (HFM), membrane carbonation (MC) in microalgal cultivation can be used to achieve a CTE near 100%. Due to the diurnal cycle in outdoor algal cultivation, an inconsistent CO2 demand with temperature fluctuations can cause pore wetting of the inner and outer fiber layers in composite HFMs. In addition, the presence of supersaturated O2 during high algal growth may change the gas transfer dynamics of the fibers, which can be critical when trying to selectively remove CO2 from a valuable gas such as biogas. This study evaluated fiber performance under conditions that mimic these effects by analyzing the carbon transfer efficiency (CTE), CO2 flux (JCO2), and outlet CO2 concentration compared to baseline values. Wetting of the interior fiber macropores resulted in an average 32% ± 8.3% decrease in flux, which was greater than for flooding of the outer macropores, which showed no significant change. All tests resulted in a decrease in CTE and an increase in outlet CO2. The presence of elevated O2 levels did not decrease the CO2 flux compared to baseline values, but it increased the O2 concentration and decreased the CH4 concentration at the distal end of the fibers. These findings highlight that liquid accumulation can decrease HFM performance during MC for microalgal cultivation, while the presence of supersaturated O2 can reduce separation efficiency.
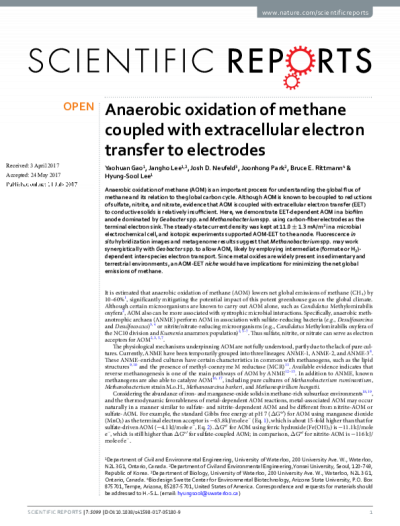
Anaerobic oxidation of methane (AOM) is an important process for understanding the global flux of methane and its relation to the global carbon cycle. Although AOM is known to be coupled to reductions of sulfate, nitrite, and nitrate, evidence that AOM is coupled with extracellular electron transfer (EET) to conductive solids is relatively insufficient. Here, we demonstrate EET-dependent AOM in a biofilm anode dominated by Geobacter spp. and Methanobacterium spp. using carbon-fiber electrodes as the terminal electron sink. The steady-state current density was kept at 11.0 ± 1.3 mA/m[superscript 2] in a microbial electrochemical cell, and isotopic experiments supported AOM-EET to the anode. Fluorescence in situ hybridization images and metagenome results suggest that Methanobacterium spp. may work synergistically with Geobacter spp. to allow AOM, likely by employing intermediate (formate or H[subscript 2])-dependent inter-species electron transport. Since metal oxides are widely present in sedimentary and terrestrial environments, an AOM-EET niche would have implications for minimizing the net global emissions of methane.
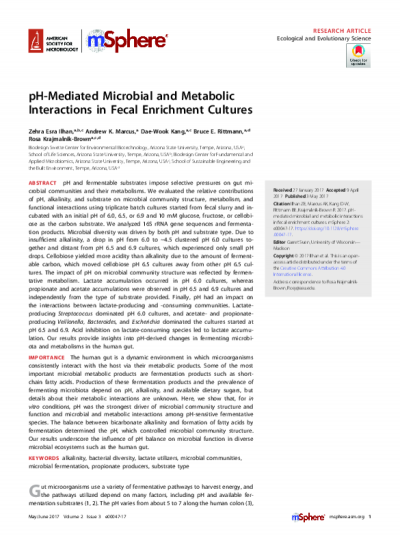
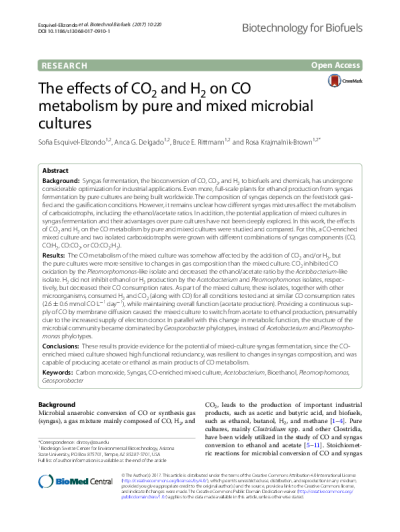
Syngas fermentation, the bioconversion of CO, CO[subscript 2], and H[subscript 2] to biofuels and chemicals, has undergone considerable optimization for industrial applications. Even more, full-scale plants for ethanol production from syngas fermentation by pure cultures are being built worldwide. The composition of syngas depends on the feedstock gasified and the gasification conditions. However, it remains unclear how different syngas mixtures affect the metabolism of carboxidotrophs, including the ethanol/acetate ratios. In addition, the potential application of mixed cultures in syngas fermentation and their advantages over pure cultures have not been deeply explored. In this work, the effects of CO[subscript 2] and H[subscript 2] on the CO metabolism by pure and mixed cultures were studied and compared. For this, a CO-enriched mixed culture and two isolated carboxidotrophs were grown with different combinations of syngas components (CO, CO:H[subscript 2], CO:CO[subscript 2], or CO:CO[subscript 2]:H[subscript 2]).
Results
The CO metabolism of the mixed culture was somehow affected by the addition of CO[subscript 2] and/or H[subscript 2], but the pure cultures were more sensitive to changes in gas composition than the mixed culture. CO[subscript 2] inhibited CO oxidation by the Pleomorphomonas-like isolate and decreased the ethanol/acetate ratio by the Acetobacterium-like isolate. H[subscript 2] did not inhibit ethanol or H[subscript 2] production by the Acetobacterium and Pleomorphomonas isolates, respectively, but decreased their CO consumption rates. As part of the mixed culture, these isolates, together with other microorganisms, consumed H[subscript 2] and CO[subscript 2] (along with CO) for all conditions tested and at similar CO consumption rates (2.6 ± 0.6 mmol CO L[superscript −1] day[superscript −1]), while maintaining overall function (acetate production). Providing a continuous supply of CO by membrane diffusion caused the mixed culture to switch from acetate to ethanol production, presumably due to the increased supply of electron donor. In parallel with this change in metabolic function, the structure of the microbial community became dominated by Geosporobacter phylotypes, instead of Acetobacterium and Pleomorphomonas phylotypes.
Conclusions
These results provide evidence for the potential of mixed-culture syngas fermentation, since the CO-enriched mixed culture showed high functional redundancy, was resilient to changes in syngas composition, and was capable of producing acetate or ethanol as main products of CO metabolism.
The ASU School of Dance presents Undergraduate Projects Showing, October 25-26, with works by undergraduate dance students, performed at Margaret Gisolo Dance Studio.
The ASU School of Dance presents School of Dance LIVE!, September 7-9, with works by dance faculty, performed at Galvin Playhouse.